In a special, dust-free, clean laboratory, straddling the Swiss-French border, a group of physicists spend their time probing hand-sized hexagons of silicon. These hexagons are a fraction of a millimetre thick and are made up of over a hundred smaller hexagons, individual sensors each roughly one centimeter across. Together with layers of metal, the sensors will form a new subdetector to replace part of the end-cap calorimeters in CERN’s CMS experiment.
A calorimeter measures the energy a particle loses as it passes through. It is usually designed to stop entirely or “absorb” most of the particles coming from a collision. The new calorimeter sensors will be used to measure the energy and arrival time and to trace the path of individual particles that fly out in the form of debris from the collision point in the centre of the experiment. Once in place, this will be the first time that this type of silicon sensor has ever been used in the calorimeter of a particle detector on such a large scale.
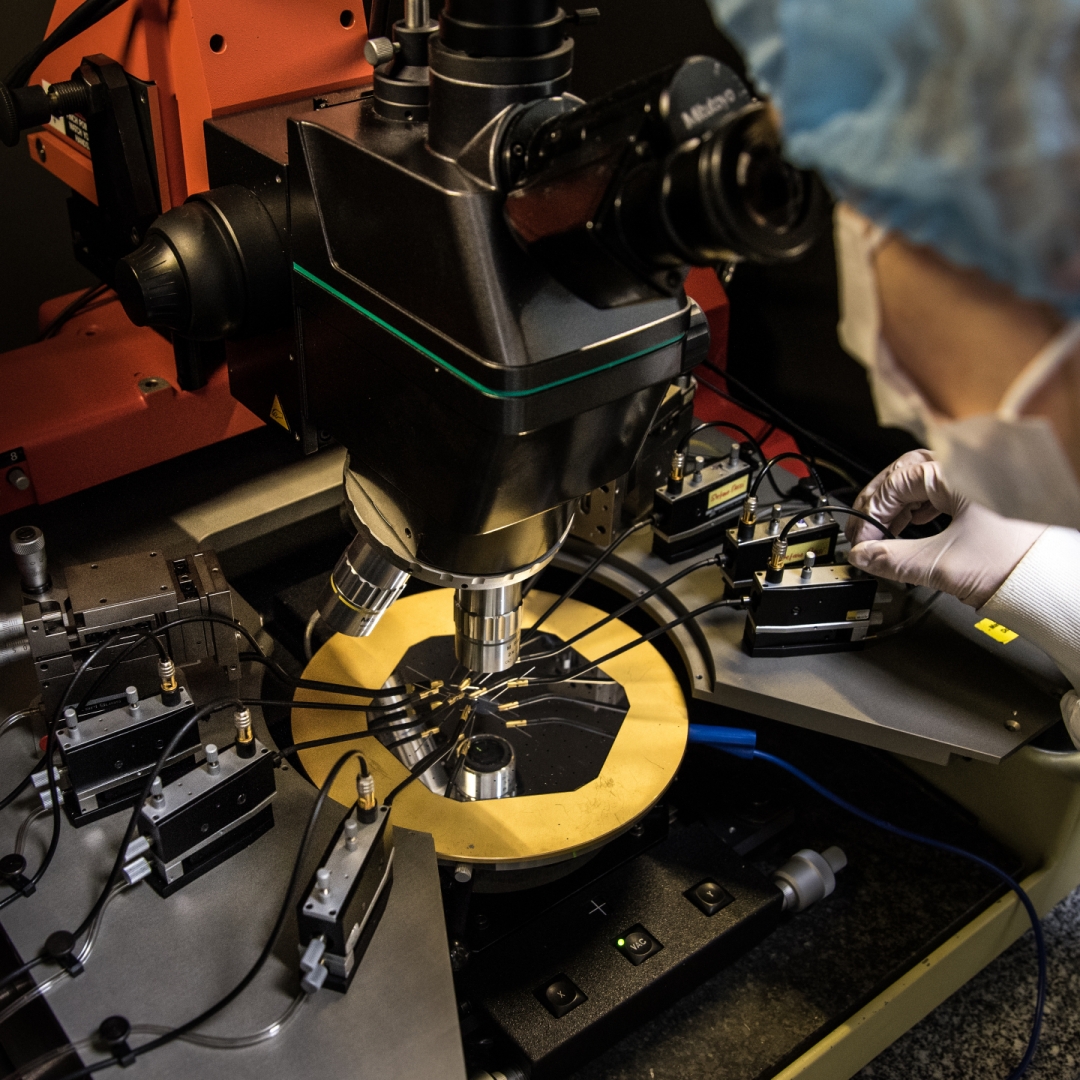
The sensors are part of a wider upgrade project to make sure that the experiments are able to cope with a larger number of particle collisions as a result of the High-Luminosity LHC (HL-LHC) upgrade in 2025, and the increased potential for discovery that comes with it. The current technology is based on long, clear lead-tungstate crystals designed to cope with the radiation in the detectors Although they will operate fine for the LHC era, until 2025, the amount of radiation expected during HL-LHC will darken the crystals until they become blind to particles passing through them.
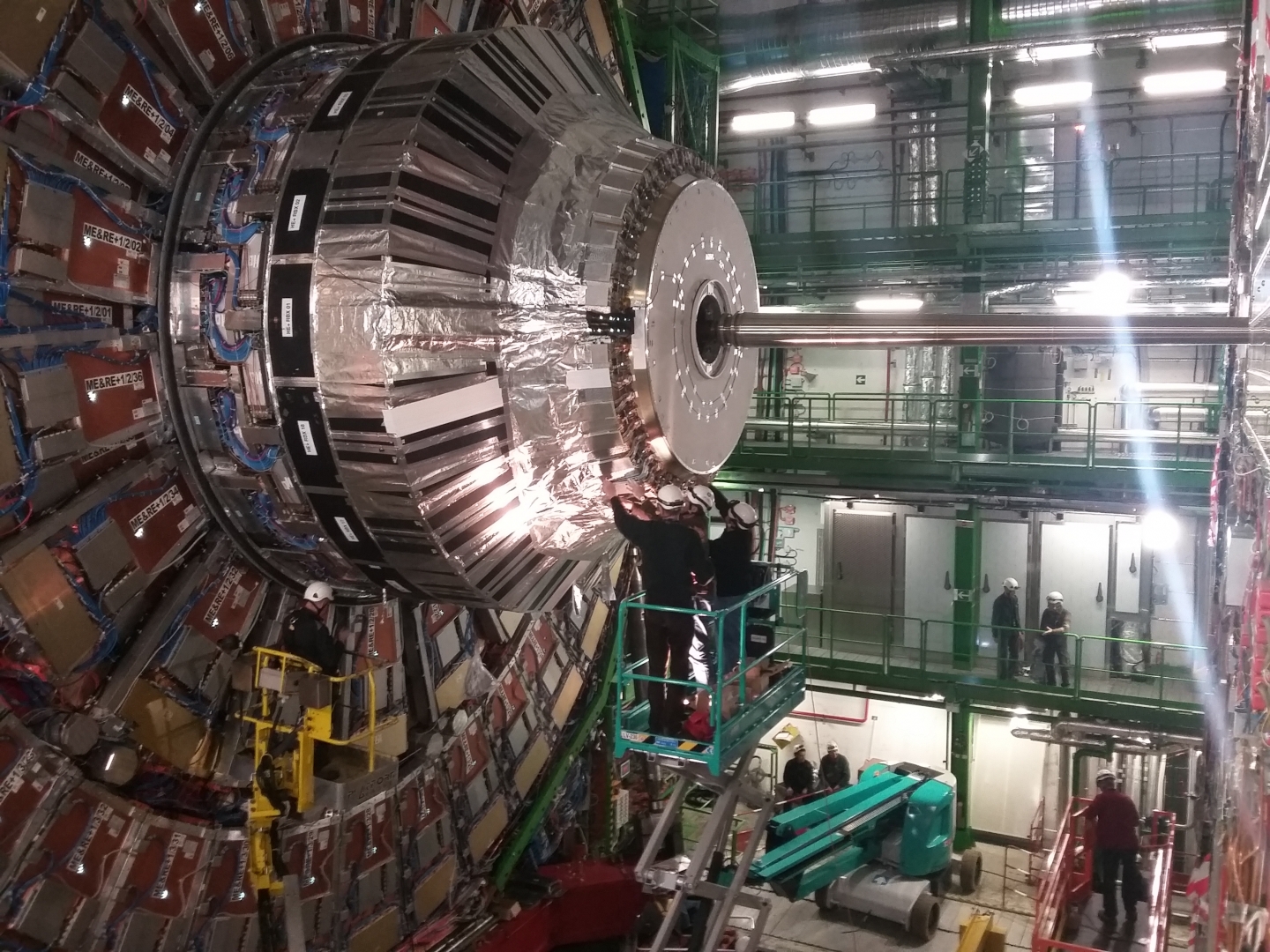
“The lead-tungstate crystals we use now are designed to operate at comparably low collision rates and in a low-radiation environment. With the HL-LHC, we’ll have hundreds of collisions at one time, so we needed something that could withstand the increased radiation and resolve showers from particles very close to each other in space and time,” explains Eva Sicking, the applied physicist leading this silicon sensor project. “We want to be able to distinguish the different particles that we see, and also know which ones came from which collisions.”
“These sensors not only provide a system that’s more radiation-hard; at the same time they also provide more information on where exactly the particles passed through. They also give us very good timing information, so we can determine exactly when this particle arrived, and thanks to the small cells it can do that for many collisions at the same time,” continues Andreas Maier, who is also working on the project.
Metal Sandwiches
To make sure the sensors are able to do this, instead of long crystals, the team are moving away from long crystals and instead building sandwiches – layers of the sensor alternating with layers of a heavy metal, such as lead.
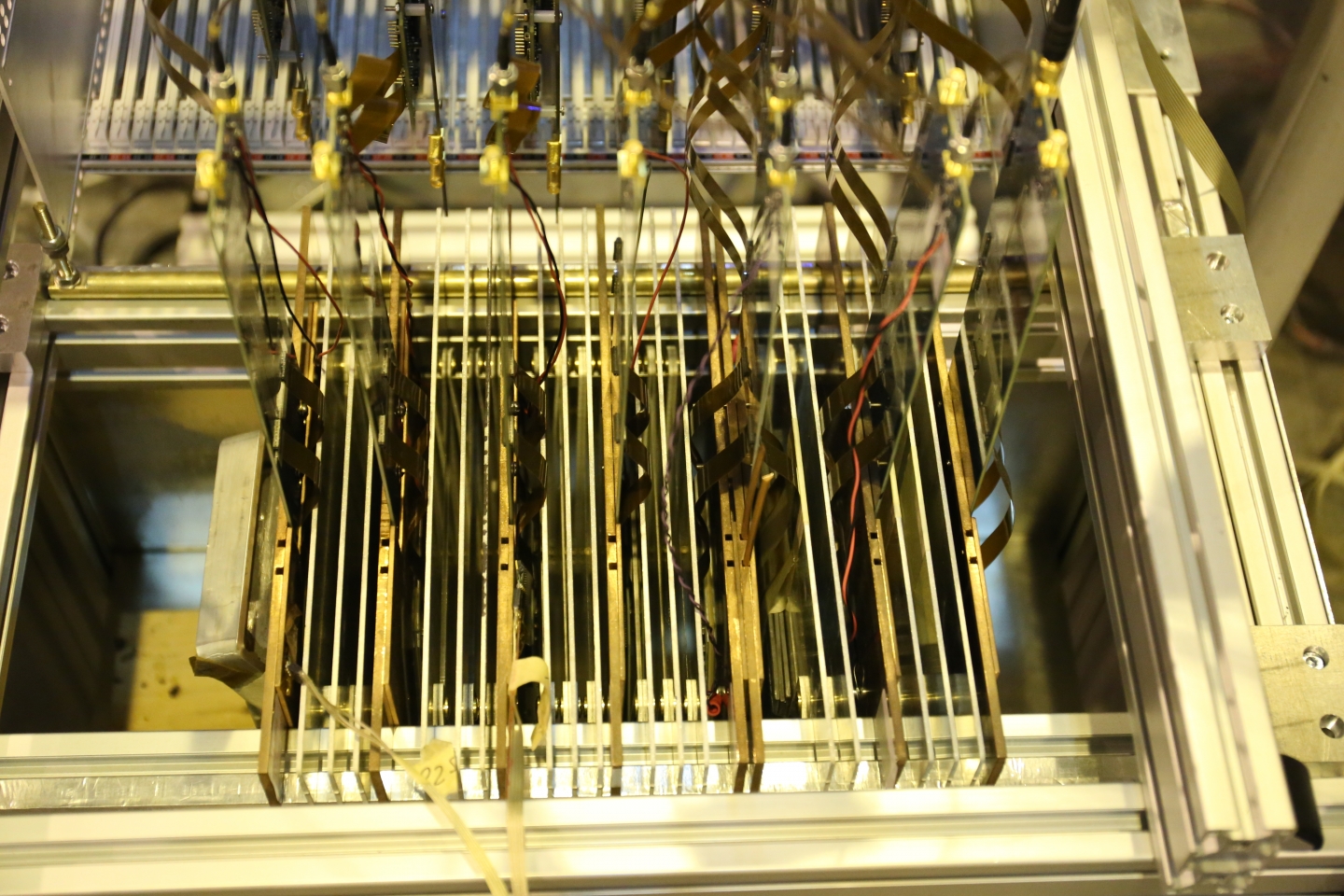
To test each sensor in the sandwich, the team is using a special probe station, with eight needles sitting above a vacuum plate. The plate holds the delicate, and expensive, silicon sensors firmly in place so that the needles can be manoeuvred and lowered to connect with contact pads marked on each sensor. They then apply a high voltage to the sensor to record the data that will be used to assess the sensor’s quality.
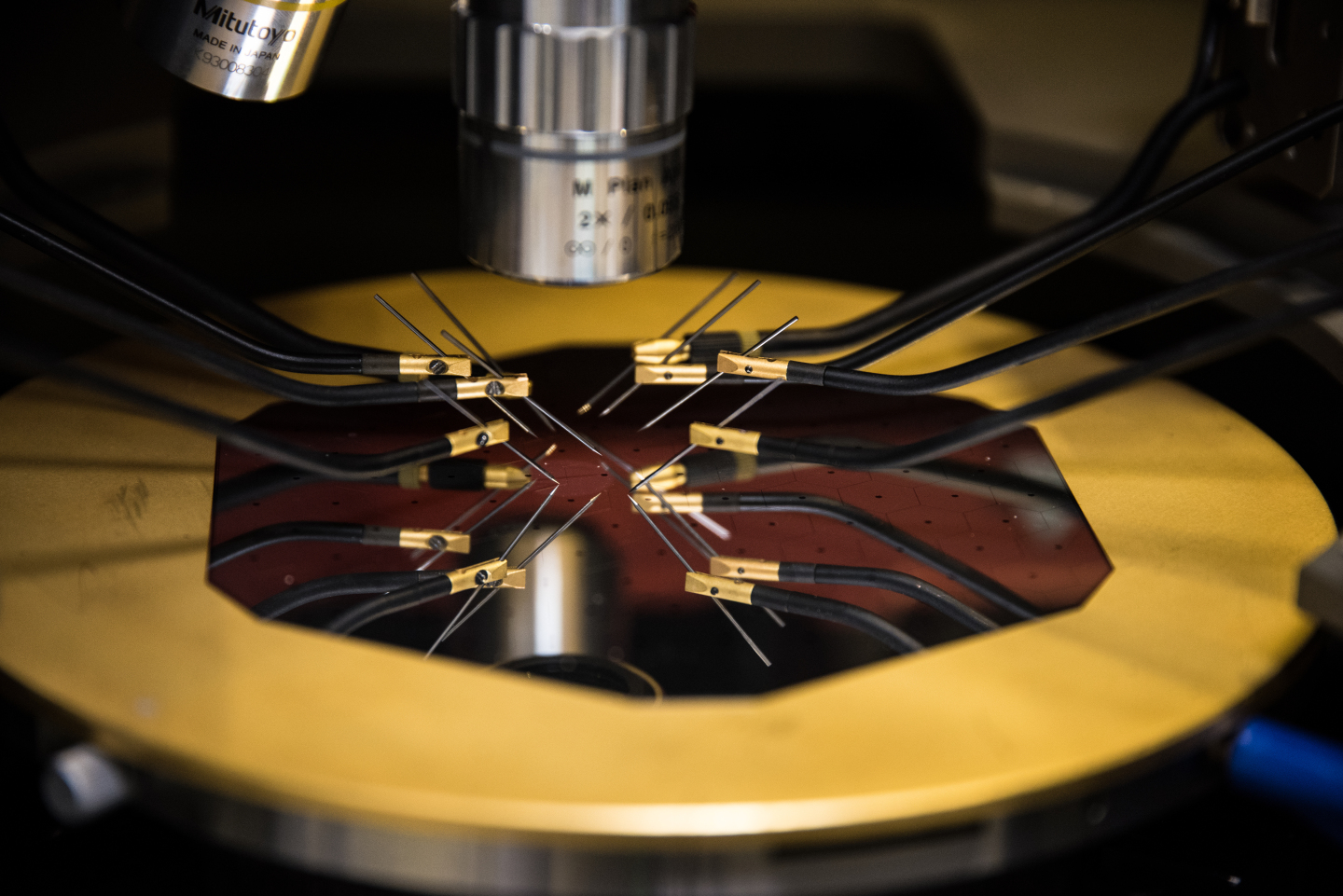
Sensitive instruments tell the team what the electrical current generated in the sensor is, as well as a measure called capacitance. If either of these run above a set level, the sensor cannot be used, as it will create noise that interferes with the data from any particle tracks. If the noise is too high, the researchers can assess if there is a problem at production level. If a problem is found, they go back to the manufacturers to make sure it’s ironed out before the real sensors go into production. All the sensors eventually used will go through this process, either at CERN or at other institutes.
Optimising power
Measuring current is particularly important because it can have an impact on how much power and energy is required when the machine is running.
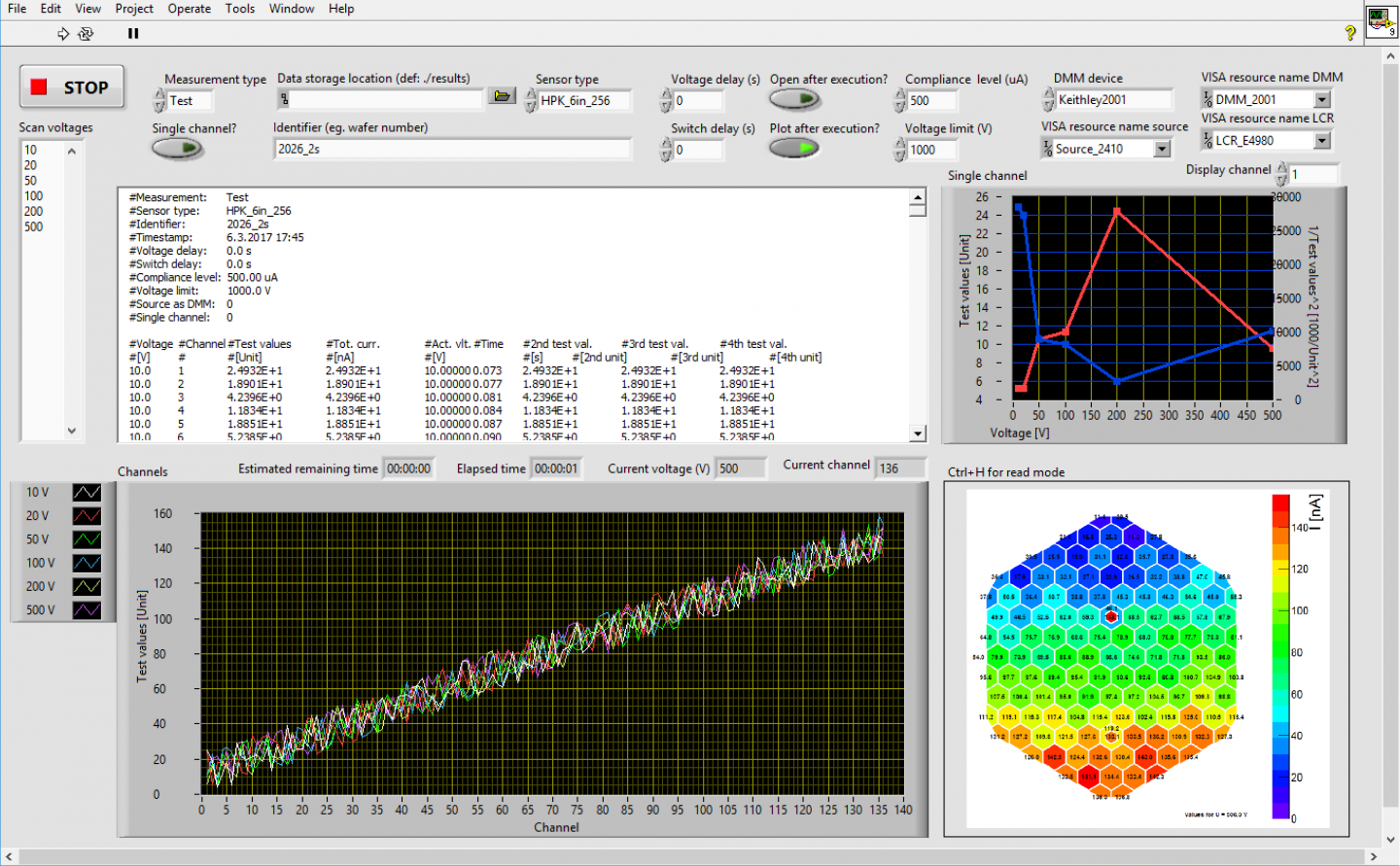
“In an ideal world, the sensor would not show any leakage current, but in reality, impurities are introduced during the production of these sensors. Therefore, the current we measure is an indicator of the production quality,” Florian Pitters, another member of the group, explains.
Leakage current is acceptable below a certain level, but it is amplified as you add more sensors together and the power supply and cooling system has to deal with a larger amount of power and dissipated heat.
“Approximately 25% of the final electricity bill will be due only to leakage current. So if we can suppress it, that’s good.” Andreas
If there’s any problem in the final sensors, it could cause the entire tile to short, rendering it useless. So these tests are vital to ensuring that the whole detector system runs at its best and that these components don’t create barriers to future discovery.
“There have been mistakes with things that people just couldn’t have known, until we tested them. We’ve discovered a few times that ways we intended to go just had to be abandoned, so we chose a new path. That’s how research goes,” says Andreas.