Subatomic particles are far too tiny to see, so over the years physicists have devised ingenious ways to detect and visualise them, often forming beautiful patterns and pictures in the process. From early experiments with cloud chambers to state-of-the-art animations of Higgs-boson decays, data visualisation in particle physics has come a long way. Here are just a few of the most striking images of particle interactions - or "event displays" - from over the years.
Cloud chambers
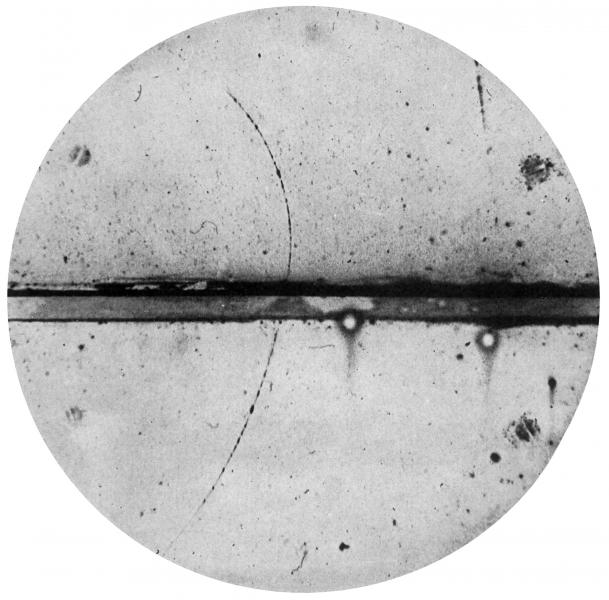
Some detectors can reveal subatomic particles by making their tracks visible to the naked eye. The first such detector was the cloud chamber, developed in 1911 by Charles Thomson Rees Wilson in Cambridge, UK – an invention for which he received the 1927 Nobel prize in physics.
A cloud chamber is a box containing a supersaturated vapour. As charged particles pass through, they ionise the vapour, which condenses to form droplets on the ions. The tracks of the particles become visible as trails of droplets, which can be photographed. During the first half of the 20th century, experiments that looked at cosmic rays passing through cloud chambers revealed the existence of several fundamental particles, including the positron, the muon and the first strange particles.
Today at CERN, the Cosmics Leaving Outdoor Droplets (CLOUD) experiment uses a special cloud chamber to study the possible link between galactic cosmic rays and cloud formation. The CLOUD chamber is used both to grow the aerosol particle seeds for cloud droplets and also to form the clouds themselves. "CLOUD uses the same principle of adiabatic cooling of humid air as in the original Wilson cloud chamber," says Jasper Kirkby of the CLOUD experiment. "But the conditions are chosen to reproduce natural clouds, involving only small water-vapour supersaturations, so particle tracks do not form."
Cloud chambers can also be found at CERN's S'Cool LAB, where students build their own devices to see how charged particles can form droplets in the vapour.
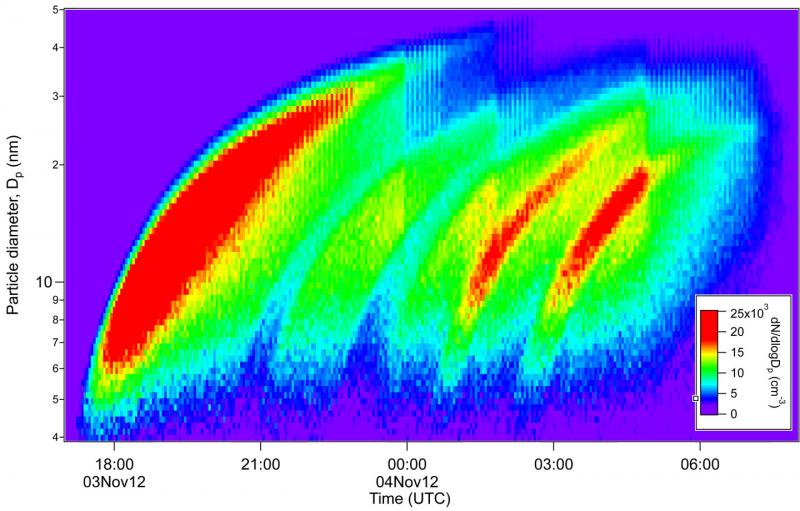
Bubble chambers
After World War II, as higher-energy particle accelerators became available, the cloud chamber was gradually replaced by the bubble chamber. Donald A. Glaser invented the bubble chamber 1952, for which he was awarded the 1960 Nobel prize in physics. It works on a similar principle to the cloud chamber, but the tracks are made visible as a trail of bubbles in a superheated liquid that is about to boil rather than in a vapour. CERN's famous bubble chamber Gargamelle was instrumental in the 1973 discovery of weak neutral currents. The discovery confirmed the prediction of such currents by electroweak theory, which treated the weak force and the electromagnetic force as different facets of the same interaction.
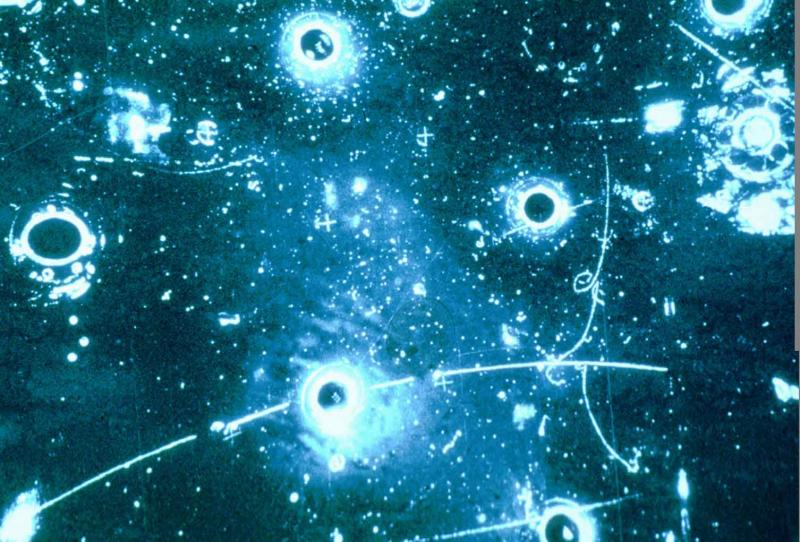
Bubbles of data
In cloud and bubble chambers, the data acquisition and event display were practically the same. Other (non-visual) particle detectors triggered cameras to take photographs in the chamber, and these were later projected onto a special table for analysis. At CERN in the 1960s, people worked in shifts round the clock to analyse such images, sifting through many thousands to find the events that physicists found interesting. They then measured the length and direction of the interesting particle tracks.
Watch people at work at a scanning table starting from 3:00 in this documentary about Gargamelle
However, a bubble chamber is sensitive to particles passing through only when its contents are superheated after rapid expansion. Bubbles form at this point and the chamber must be recompressed to stop the bubble growth for a picture. This limits the rate at which events can be collected. For instance, the huge Big European Bubble Chamber (BEBC), which started operation at CERN in 1973, took 6.3 million pictures during its 11 years of service. Current experiments at the Large Hadron Collider (LHC) record this number of events in less than 2 hours.
The spark chamber improved on the bubble chamber as interactions could be captured much more rapidly. In a spark chamber, particles pass through an inert gas such as neon, forming tracks. A voltage is applied to plates on alternate sides of the chamber, causing a trail of sparks to flash across the gas.
Charpak and the move to digital
Though spark chambers were faster than bubble chambers, they did not provide the detail – the resolution – that a bubble chamber could.
In 1968 at CERN, the French physicist Georges Charpak developed the “multiwire proportional chamber” to overcome the limitations of spark chambers, both in speed and their resolution. Charpak's chamber was basically a gas-filled box with a large number of parallel detector wires, each connected to individual transistor amplifiers. Now there was no need for a spark; a detector wire connected to an amplifier can detect a much smaller effect. Linked to a computer, Charpak’s chamber could achieve a counting rate a thousand times better than existing detectors.
Charpak's invention, for which he received the 1992 Nobel prize in physics, revolutionized particle detection. It made data acquisition quick, automated and electronic. As a result, it also changed the nature of event displays.
Photographs were no longer the only way to visualise particle tracks in detail; rather, event displays became visual representations of patterns of digital signals that corresponded to the particles produced in an interaction. Moreover, the event display can be made to show only those tracks that physicists find interesting. So the display has become a visual representation of the most interesting part of what happened in the detector.
As detectors became more complex and able to detect many more particles at a time, the amount of data associated with each event increased and event displays became correspondingly more intricate. Researchers developed software that could interpret the patterns of signals picked up by detectors and recreate them as images in 3D space.
The advent of computer colour screens in the late 1970s allowed physicists for the first time to render event displays in full colour, leading to discussions about which were the most suitable hues to represent different particles. Coupled with computer systems such as the Megatek, these displays could even be manipulated in 3D.
Digital data
Tom McCauley of the University of Notre Dame in the US makes event displays for the CMS experiment at CERN. "The days when data acquisition and event display were practically the same are no more," he says. "The LHC produces hundreds of millions of proton-proton collisions per second, which produce very complex events, and the detectors are correspondingly sophisticated. The displays reflect this complexity but are useful since they can provide a visual summary of what happened; you can describe geography and a route with words, but sometimes nothing beats a map with a line marking the way."
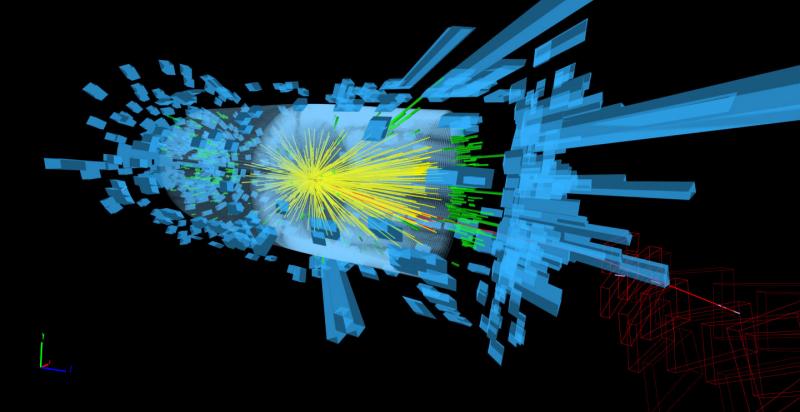
These days to create a display, experiment teams run software that converts the data into graphical objects. These graphics are then rendered in a specialised application. The details of the display – views, colours, what is shown and what is not – depend on the particular use-case.
Physicists at CERN use event displays for viewing geometry, developing algorithms and detector monitoring. The displays are also frequently used in communicating LHC science to the general public and to the media. And the images continue to become increasingly detailed.
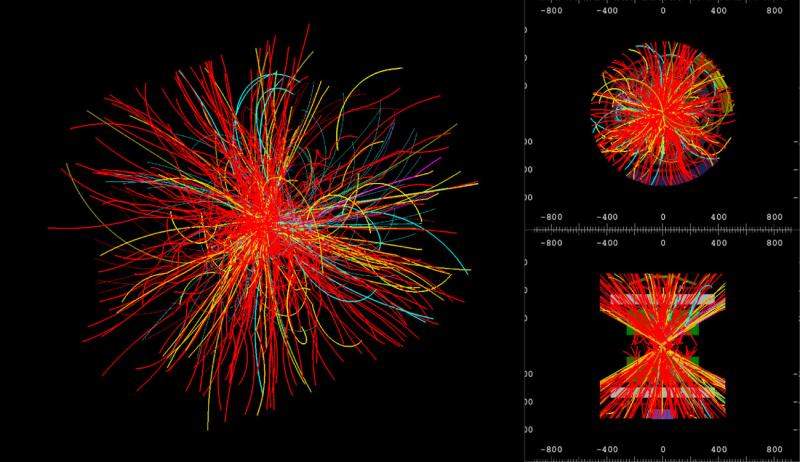
"These days thanks to advances in computing we're capable of so much more graphics-wise and can run on many different devices and platforms," says McCauley. "I find it amazing that today I can run an event-display application on my phone!"
The nature and complexity of event displays, and even how they are generated in the first place, have changed considerably since Wilson's first cloud-chamber photos in 1911. But one thing has not changed.
"Conveying the physics accurately is always the primary consideration," says McCauley.