The High-Luminosity LHC (HL-LHC) is a major upgrade of the Large Hadron Collider (LHC). The LHC collides tiny particles of matter (protons) at an energy of up to 14 TeV in order to study the fundamental components of matter and the forces that bind them together. The High-Luminosity LHC will make it possible to study these in more detail by increasing the number of collisions by a factor of between 5 and 7.5 with respect to the nominal LHC design.
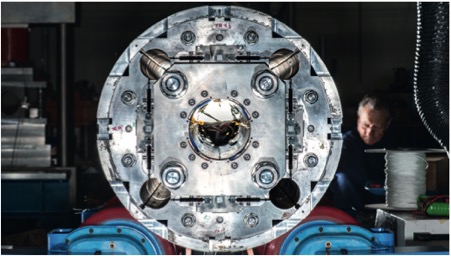
Prototype of a quadrupole magnet for the High-Luminosity LHC. (Image: Robert Hradil, Monika Majer/ProStudio22.ch)
What is luminosity?
Instantaneous Luminosity, which is the measure of the number of potential collisions per surface unit (or cross section) over a given period of time, is an essential indicator of an accelerator’s performance. Integrated luminosity is a measure of the collected data size and defined as the time integral of the instantaneous luminosity measured in inverse femtobarns (fb−1). Assuming the nominal total proton cross section at the LHC at 7 TeV of 100 mbarn, one inverse femtobarn of integrated luminosity equates to 100 million million collisions.
By the end of its first few years of operation at 13 TeV (at the end of 2018), the LHC has produced just above 160 inverse femtobarns of data. The HL-LHC will produce more than 250 inverse femtobarns of data per year and will be capable of collecting up to 4000 inverse femtobarns during its exploitation period of 12 years.
Why High-Luminosity?
The phenomena that physicists are looking for have a very low probability of occurring and this is why a very large amount of data is needed to detect them. Increasing luminosity produces more data, allowing physicists to study known mechanisms in greater detail and observe rare new phenomena that might reveal themselves. For example, the High-Luminosity LHC will produce at least 15 million Higgs bosons per year, compared to around three million collected during LHC operation in 2017.
How will the High-Luminosity LHC work?
Increasing the luminosity means increasing the number of collisions: at least 140 collisions will be produced each time two particle bunches meet at the heart of the ATLAS and CMS detectors, compared to around 40 collision events at present. To achieve this, the beam will need to be more intense and more focused than at present in the LHC. New equipment will need to be installed over about 1.2 of the LHC’s 27 kilometres.
- More powerful focusing magnets and new optics
New, more powerful superconducting quadrupole magnets will be installed on either side of the ATLAS and CMS experiments to focus the particle bunches before they meet. These magnets will be made of a novel superconducting compound, based on niobium and tin [Nb3Sn], used for the first time in an accelerator, which will make it possible to achieve higher magnetic fields than the niobium-titanium alloy used for the current LHC magnets (12 Tesla as opposed to 8). Twenty-four new quadrupole magnets are currently in production, six of which will be installed on each side of the two high luminosity experiments ATLAS and CMS. The use of niobium-tin magnets is also an opportunity to develop this technology for large scale application in future accelerators.
New beam optics (the way the beams are tilted and focused) will notably make it possible to maintain a constant collision rate throughout the time the beams will collide.
- “Crab cavities” for tilting the beams
The particles circulate in the LHC in distinct packages, called bunches. Each bunch is about 30 cm long. This innovative superconducting equipment will give the particle bunches a transverse momentum before they meet, enlarging the overlap area of the two bunches and thus increasing the probability of collisions. A total of sixteen crab cavities will be installed on both side of each of the ATLAS and CMS experiments.
- Reinforced machine protection
As the beams will contain more particles and hence almost twice the stored beam energy of the current LHC, machine protection will need to be reinforced. Around one hundred new, more performant collimators will be installed, replacing or supplementing the existing ones. These devices absorb particles that stray from the beam trajectory and might otherwise damage the machine.
- Crystal collimators
New crystal collimators will be installed in the dedicated cleaning insertions to improve the cleaning efficiency of the insertions for ion beam operation. Without these devices, the higher ion beam intensities would result in unacceptably high losses in the superconducting magnets.
- Innovative superconducting links
Innovative superconducting power lines will connect the power converters to the new accelerator magnets. These cables, which are around one hundred metres long, are made of a novel superconducting material, magnesium di-boride, that is superconducting at a much higher temperature than the magnets, and can therefore be cooled more energy-efficiently by using gaseous Helium. They will be able to carry currents of record intensities, up to 100 000 Amps when being cooled down to temperatures below 50 Kelvin!
- An upgraded accelerator chain
The HL-LHC’s performance will also rely upon the injector chain, i.e. the four machines that pre-accelerate the beams before sending them into the 27-kilometre LHC ring. This accelerator chain has been upgraded during the second long shutdown of the LHC between 2018 and 2021.
What is the work schedule?
In order to install the new equipment and move certain components around, new underground structures and surface buildings are required.
The civil engineering work began in April 2018 at LHC Point 1 (in Meyrin, Switzerland), where the ATLAS experiment is located, and at LHC Point 5 (in Cessy, France), the site of the CMS experiment. A new shaft of around 80 metres, as well as an underground cavern and a 300-metre-long service tunnel has been dug on each site. This service tunnel is linked to the LHC tunnel by four connecting tunnels and 12 vertical cores for each of the two high luminosity experiments ATLAS and CMS. The four main connections between the new and old infrastructures have already been established in 2019. Five surface buildings will be built on each site to house the electrical, cryogenic and cooling and ventilation infrastructure of the new HL-LHC equipment.
In the meantime, the new equipment is being manufactured in Europe, Japan, the United States and China. Canada will also contribute to the production of the state-of-the-art equipment. The experiments are also preparing for major upgrades of their detectors to deal with the deluge of data promised by the HL-LHC.
Installation of the first components (the crystal collimators, beam instrumentation, some other collimators and new shielding) has taken place during the second long shutdown of the LHC. Most of the equipment and the major experiment upgrades will however be installed during Long Shutdown 3, to take place between 2026 and 2028.
How much will the High-Luminosity LHC cost?
The material budget for the accelerator is set at about 1 billion Swiss francs between 2015 and 2028, including many in-kind contributions from international partner laboratories.
Who is involved in the project?
CERN and its Member and Associate Member States are supported by an international collaboration of 43 institutions in 19 countries, including the United States, Canada, Japan and China.
How will society benefit from the HL-LHC?
The HL-LHC will further our fundamental knowledge, which is CERN’s primary mission. To develop the HL-LHC upgrade, CERN is pushing several technologies to new limits, such as electrical engineering, notably in terms of superconductors, vacuum technologies, computing, electronics and even industrial processes. In the long term, these innovations will benefit our daily lives.
For example, superconducting magnets find applications in the fields of medical imaging and cancer treatment with particle beams (hadron therapy). There are also many prospects in the field of electrical engineering: European industry is studying the possibility of using magnesium di-boride cables to transport high electrical power over great distances in a way that is sustainable for the environment.
The HL-LHC project is also contributing to the training of new scientists – physicists, engineers and technicians. Currently, more than 200 bachelor and master students, doctoral students, post-doctoral researchers and fellows of 23 different nationalities are participating in the project.
--
HL-LHC brochure (a printable version of the text shown above)
CERN press release, 15 June 2018: Major work starts to boost the luminosity of the LHC